Seeing as it has taken me quite some time to post this, you’re probably all tired of playing on Chess on the non-Euclidean boards that I described previously. Which means that it’s time to explore Roice Nelson’s idea of hyperbolic chess.
First, lets talk a little about 2D hyberbolic tilings for those who haven’t encountered them in the past. On a Euclidean plane, we can tile squares onto a checkerboard such that four corners meet at a point.
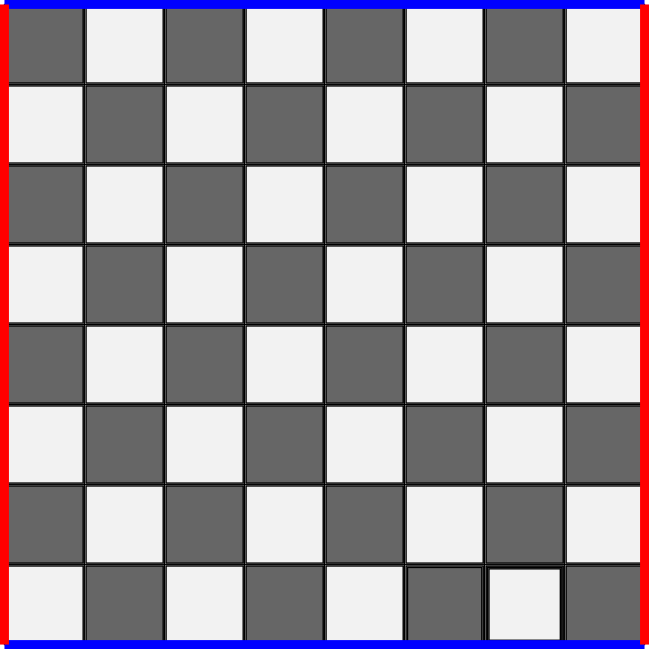
On a hyperbolic checkerboard more than 4 regular quadrilaterals meet at a vertex when tiling a plane. This means each angle of a square must be less than 90 degrees (if 5 squares meet at a vertex in a planar tiling, then the angles should be 360/5 or 72 degrees).
There are lots of potential regular hyperbolic tilings that we could imagine playing chess on. We’d like to preserve the checkerboard property of our tiling, so we will only consider tilings where an even number of regular polygons meet at a vertex. Here are a few potential tiling candidates drawn using the Poincaré disk model*.
The {4,6} hyperbolic tiling is a tiling of 4-sided polygons (squares) such that 6 of them meet at a vertex. It’s nice because it keeps the shape of the tiles in the checkerboard as squares.
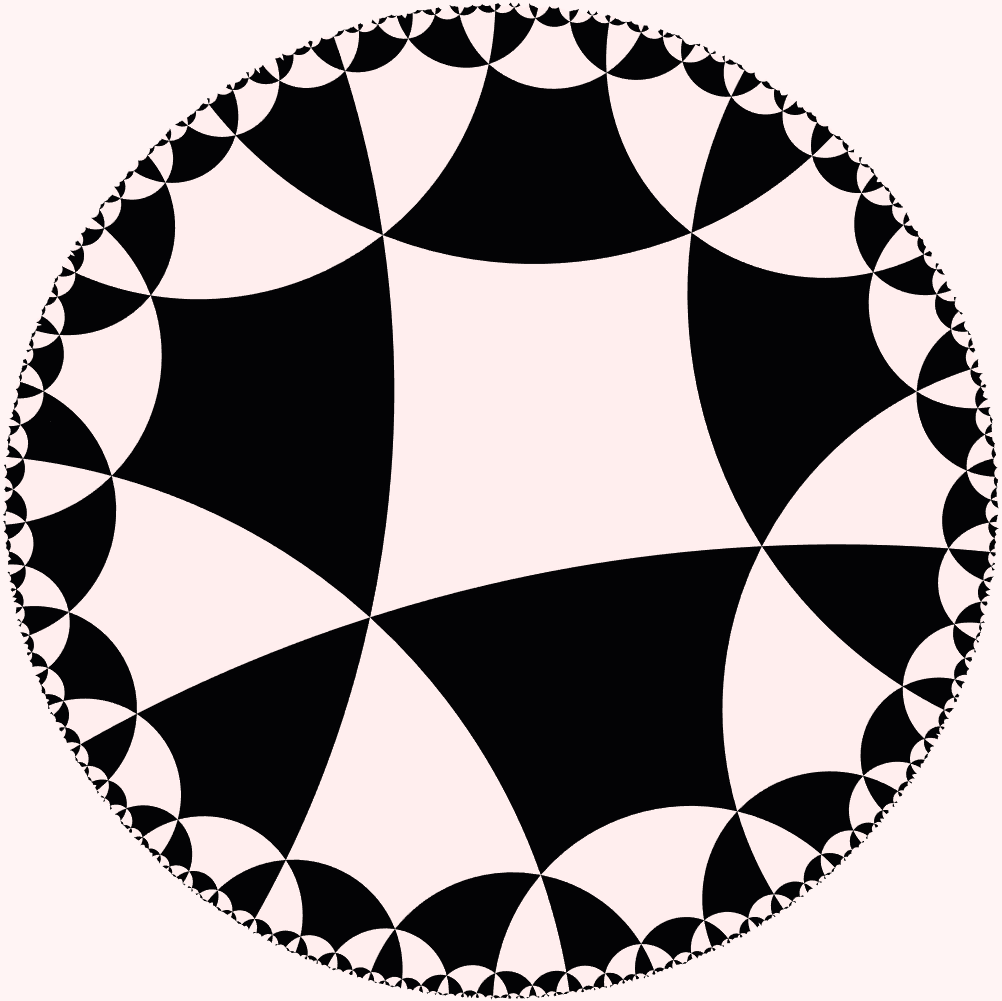
A {5, 4} tiling where 4 pentagons meet at a vertex maintains the property that a piece moving along the diagonal can only visit tiles of one of the two colors. On the other hand, it becomes extremely difficult to define the opposite side from a side or the opposite corner from a corner, so moving in a straight line quickly gets messy.
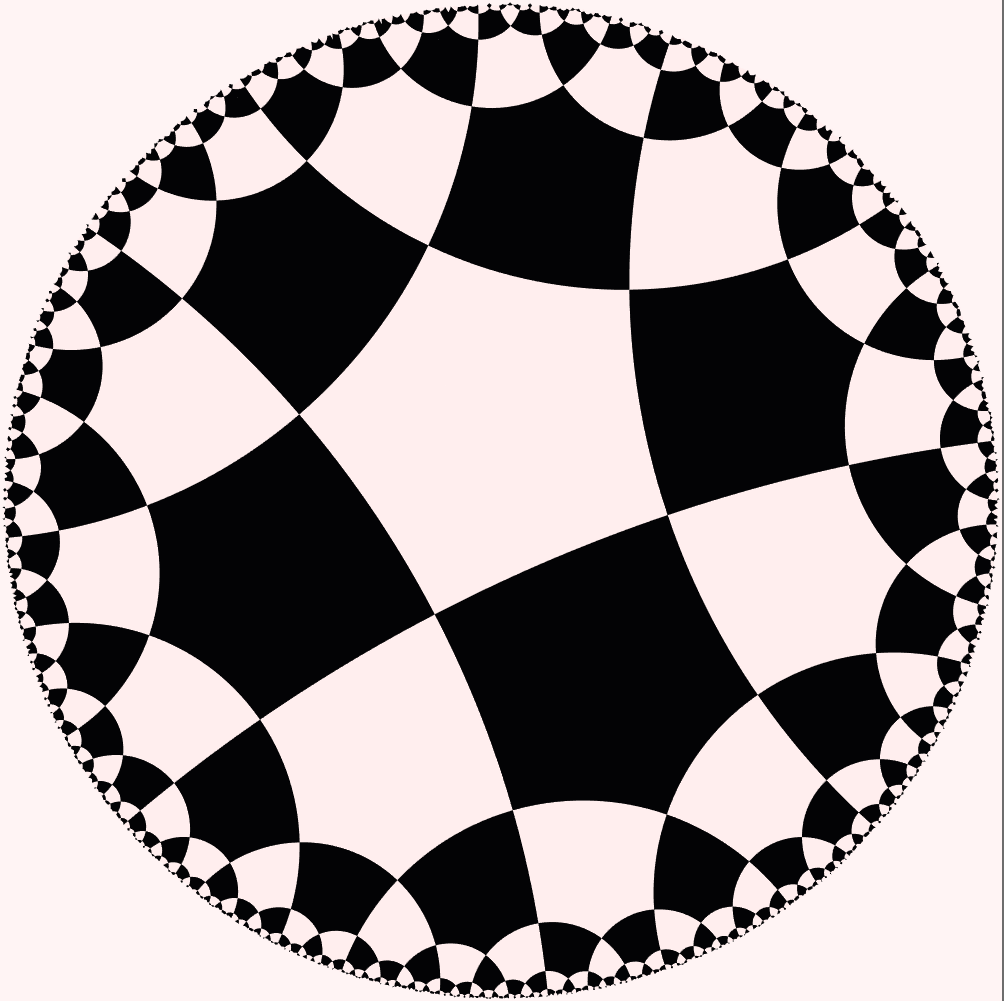
Finally, the {6, 4} and {4, 8} tilings start to get pretty busy, but do nicely maintain the idea of having opposite edges well-defined (lacking in a pentagonal tiling), while keeping opposite corners colored the same.
The first issue that we face with our hyperbolic chess board is that it isn’t going to lie flat in 2D Euclidean space, so pieces are going to want to slide off (thanks, gravity!). Thus, playing hyperbolic chess with a physical board requires either that our pieces actually stick to our board (velcro?), that we put up with the large amount of square distortion that occurs when trying to embed a hyperbolic board on a Euclidean plane, or that we write and play our game on a computer.
Looking at the tiling candidates above, the {4, 6} tiling and other square tilings seem like clear winners for our hyperbolic chess game. In particular, it is immediately obvious what it means to travel horizontally and vertically across it.
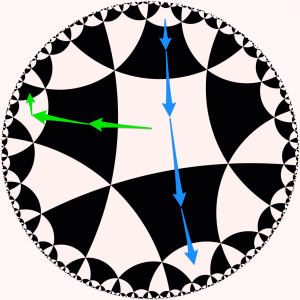
The main question is what it means to travel diagonally across the tiling, when leaving a corner of the {4,6} tiling there is one obviously diagonal square, and two still kind of diagonal squares adjacent to it. However, the obviously diagonal square doesn’t share a color with the original square, which means that we lose one of the standard rules of chess – that each bishop can only visit a single color of square. It also more or less ruins the game of checkers, in my opinion. Moreover, if we only allow the “obvious” diagonal, then a bishop would be unable to reach a majority of the squares in the tiling. Therefore, I instead define a ‘reasonable diagonal’ to a square, A, as any square, B, that shares a vertex and a color to A.
For checkers it seems reasonable to allow going to either of them at every diagonal. This seems to be in keeping with the spirit of the game and to be likely to keep game play reasonably balanced.
It’s less clear that this is a good strategy for chess. It certainly makes the pieces that can travel along diagonals much more powerful, as they can now travel to many more squares than ones that can only travel horizontally or vertically. That said, my guess is that a reasonable strategy would be for pieces moving along the diagonal to be able to make a choice when moving out the very first corner to either take the “right” or “left” path and to always take that path on any given move. Thanks to the negative curvature of the board and the straight lines we are requiring, this does leave an interestingly large space that it would take any given piece multiple turns to reach.
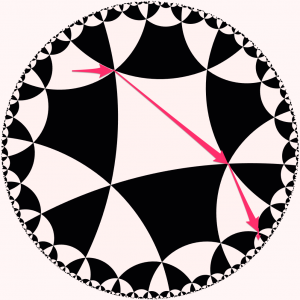
Another question is how big to make the surface and where to position the pieces at the start of the game.
Since the horizontal and vertical distances are pretty well defined, we can use them to define the size of the board and the location of the pieces. We start by placing the White queen on a white square. Place a white pawn in a vertically adjacent square. place the remainder of the white pawns such that they are in the same horizontal line as the white pawn you have just placed (with the same number on each side as in chess, of course). Place the remainder of the white pieces such that they are placed correctly as in chess and in the same horizontal line as the white queen. Define one edge of the board as directly behind these pieces.
From the white queen count 7 more squares vertically in the direction of the white pawns, and place the black queen there. Following similar rules to the above, place the remainder of the black pieces and define another edge of the board.
It would be nice to define sides to our board. Unfortunately, this is necessarily not as nice or easy to do as the front and back the way that we have defined the rest of the board. In chess, sides are most important for queening, which only requires the back edge, and for cornering the pieces (ie. the King during endgame). This means that defining sides isn’t strictly necessary, but we could possibly define them anyways by starting from the corner piece and constantly turning towards the center of the board to define side edges.
Pentagons are my favorite polygon, so I’m pretty fond of the {5, 4} tiling. If chess had originally been developed on a board like this, we might imagine that the standard move might involve entering a square on a face and leaving at the opposite vertex or vice versa. Perhaps rooks would have to start their move going across an edge, bishops would have to start going across a vertex, and queens could do either. Knights have the natural travel rule of having to go through an edge, then through one of the two far edges. I love the elegance and naturalness of these rules for the tiling. One might almost imagine that chess was invented for this tiling and that our Euclidean version came out of an attempt to modify the rules to fit a Euclidean chess board.
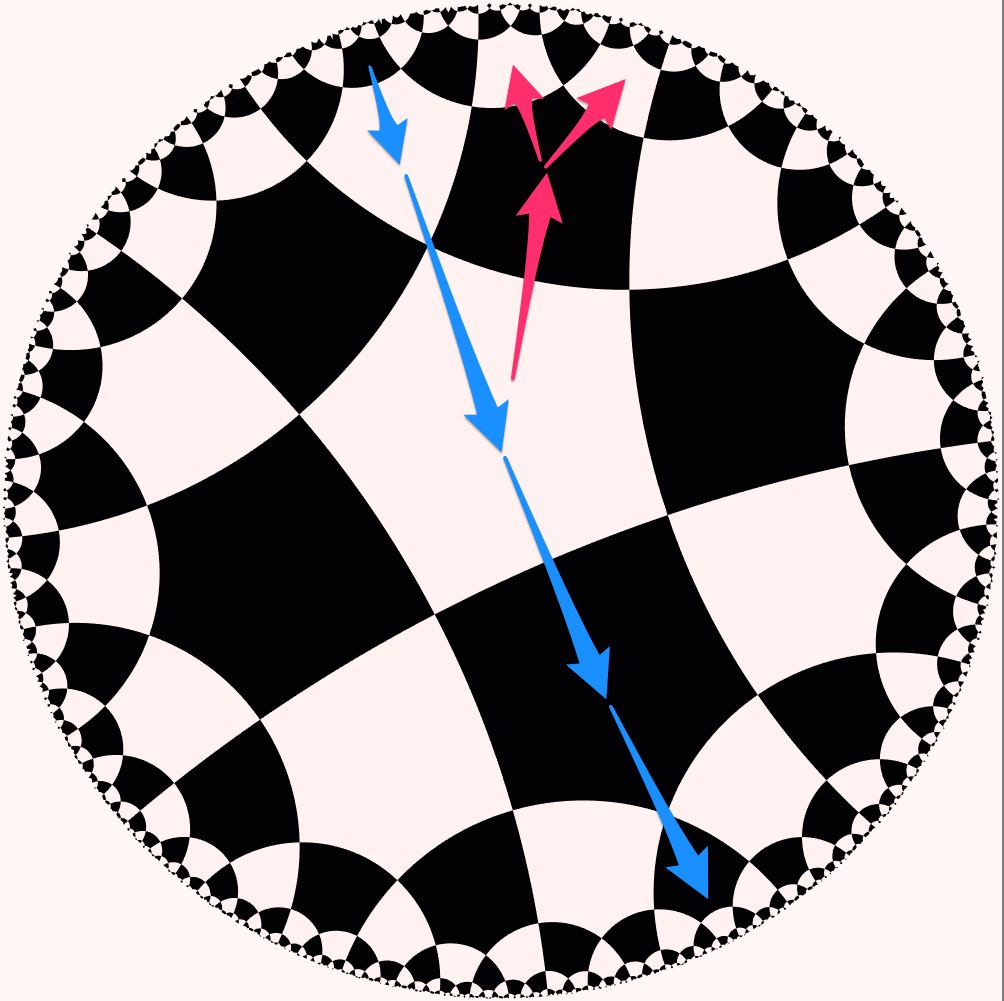
I hope you’ve enjoyed our adventures through non-Euclidean chess. It’s interesting to realize how much the apparently** Euclidean nature of our world influences the games that we play and the rules that we make for them. Rules that are straightforward in Euclidean geometry become bizarre, insufficiently well-defined or otherwise sub-optimal in other geometries and vice-versa.
* Hyperbolic tilings were created with the aid of KaleidoTile
** According to general relativity, space is curved, but we don’t notice it so much at our scale